Waste in Space. Trucking Nuclear Waste on the Space Shuttle.
Launching the By-Products of Nuclear Power into Space
The hundred years that made up the twentieth century was full of tremendous innovation and technological progress, but it was also littered with bad ideas. It is said that “necessity is the mother of invention,” and where there is a problem, someone will find a way to solve it. Of course, in some cases, the solution might be as bad or worse than the problem. That brings us to possibly the worst idea of all time, how to take the dangerous byproducts of nuclear power generation and send it packing, into space.
It started in the early seventies when the Atomic Energy Commission partnered with NASA to study the feasibility of launching nuclear waste into space. The shuttle was on the drawing board, and it seemed the ideal transport to do the job. Now you might think that this wasn’t the worst idea you ever heard on the surface, at least not at the time, but the devil indeed was in the details.
At the time of the study, a resurgence in environmentalism and ecology occurred in the United States amid global anti-nuclear protests. The timing could not have been worse to pitch the idea. Piling on was that the problem wouldn’t be solved with one launch, or two, or three launches, but hundreds. Even if you could get past that, there was the problem of once it was in space. Maybe it could come back and intercept the Earth at a future date, but not to worry, a bombardment of our nuclear waste from space was three hundred thousand years in the future, if the math was right.
The first study was undertaken at NASA’s Lewis Research Center, now the NASA Glenn Research Center, in Cleveland, Ohio. During the week of April 11, 1973, the study results were presented at the Tenth Space Congress, held at Cocoa Beach, Florida. Frank Rom, NASA’s Chief of the Power Applications and Systems Analysis branch, provided the summary. It concluded that launching nuclear waste into space could be done safely, presented a low risk, and was economically achievable.
That was the good news. On paper, it seemed safe, plausible, and affordable. Now, let’s take a look at the details on the plan more closely.
The plan looked at four destinations for the waste once it was in space.
- Earth Orbit
- Solar Orbit
- Solar System Escape
- Direct Impact into the Sun
The options of solar system escape and launching the waste directly into the sun were deemed not feasible. Launching a spacecraft towards the sun takes a significant amount of energy, and no launch vehicle in 1973 was capable of performing that type of mission. Solar system escape was equally complicated. The craft would need to use a gravity assist to get booted from the solar system, and that would add significant costs and complexity, making it untenable.
Earth orbit and solar orbit were now the remaining options. Remember, these options were long before the shuttle was built, and before an accurate assessment of its costs, capabilities, and limitations were known. The remaining options anticipated that a variety of expendable launch vehicles and the shuttle would be utilized extensively. Those expendable vehicles included the Saturn V, Atlas-Centaur, and the Titan III.
For the Earth orbit option, the thinking was that this would be a temporary solution. A more advanced Earth society would solve the disposal problem and retrieve the containers at a future date. Those containers would orbit the Earth at a distance of 34,000 miles. Well beyond the 22,236 miles of geostationary satellites, yet close enough to be retrieved and not affected by lunar perturbations.
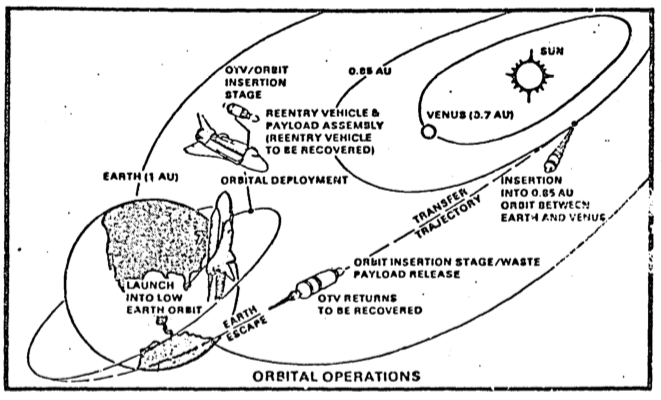
The heliocentric option planned to inject the material into an elliptical orbit that would not intercept the Earth for at least 300,000 years. That would be just long enough for the material to render itself harmless. Of course, all of this was contingent on getting the material safely into space. For that, the shuttle would be the preferred delivery mechanism. With tons and tons of material already accumulated, and with the projected expansion of nuclear power in the 80’s, the frequency of launches would be daunting.
The Space Shuttle can do it!
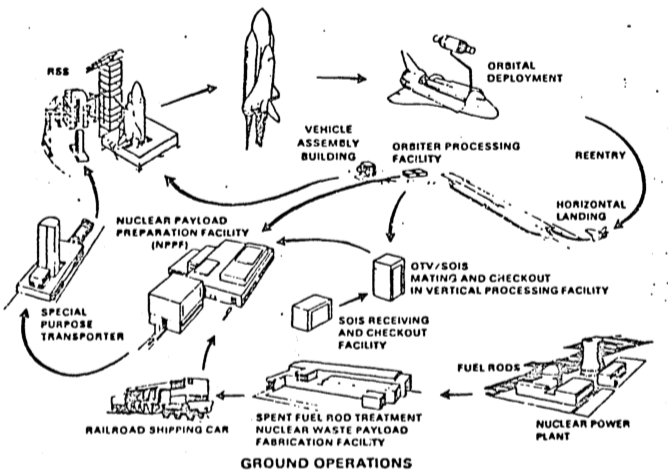
It was estimated that by the year 2000, the Space Shuttle would need to launch one hundred times a year to dispose of nuclear waste. That equates to nearly two launches per week on what was initially planned to be a five-shuttle fleet. Putting that number in perspective, over the thirty-year history of the Space Shuttle program, 135 launches were performed. The high watermark for the number of shuttle launches in a single year was nine in 1985. On average, it managed just five launches per year. Remember, each shuttle was designed for one-hundred flights. At the pace prescribed by the study, and by NASA’s original intent to have shuttles flying weekly, the entire fleet would be replaced every four years.
No one could have known eight years before the first Space Shuttle took to the skies that the promised turnaround time between flights would be weeks to months. The plan banked on the shuttle delivering on its promise of being economically superior to other launch platforms and that the shuttle would indeed fly just like a commercial airline, on a regular schedule, and of course, flawlessly.
Such a plan’s enormous costs would be funded by adding 2.5% to 5% to each home’s electric bill. It was a matter of cost versus risk. Continue to store the increasing amount of nuclear waste here on Earth with all the possible problems it posed, or permanently dispose of it in space.
Planning for the worst-case scenario
The technical challenges and risk mitigation to getting from the ground to orbit with a cargo of spent nuclear fuel were not easy—a host of contingencies and precautions needed to be considered. The first was securing the material in the right type of containment vessel while onboard the shuttle. The spent fuel was highly radioactive and generated a significant amount of heat. That would require that the payload be shielded to protect the crew, and have some form of heat dissipation mechanism. Equally important was that the design needed to account for an accident on the launch pad, during launch, or while in space.
During that same conference, it was announced that tests have shown that it was possible to create a container that could withstand a catastrophic event and maintain its integrity. One test fired the container on a rocket sled at over 715mph into a five-foot block of reinforced concrete. The test obliterated the concrete and compressed the container, but showed no structural failure evidence that would cause a leak to occur. A second test launched a container into the ground at over 750mph creating a six-foot crater. Once again, the container survived intact. It appeared that the core containment problems were resolved. The idea was far ahead of its intended ride to space. The shuttle existed only on paper, and the country was in a state of upheaval on many fronts. Then President Nixon had already cut NASA’s budget and compromised the design of the Space Shuttle. The idea was going nowhere.
The Idea Resurrected
It might have been a bad idea that wouldn’t die. The problem of nuclear waste still existed and wasn’t going away any time soon. At least for the next 300,000 years. The concept of launching nuclear waste into space was reborn in the early ’80s. This time the shuttle had been up and running for less than a year, albeit not up to the expected performance, when a new proposal was put forth. The previous Carter administration had cut the shuttle fleet by one orbiter to save costs, but there was hope that with some tweaks, spent nuclear fuel would be flying into space.
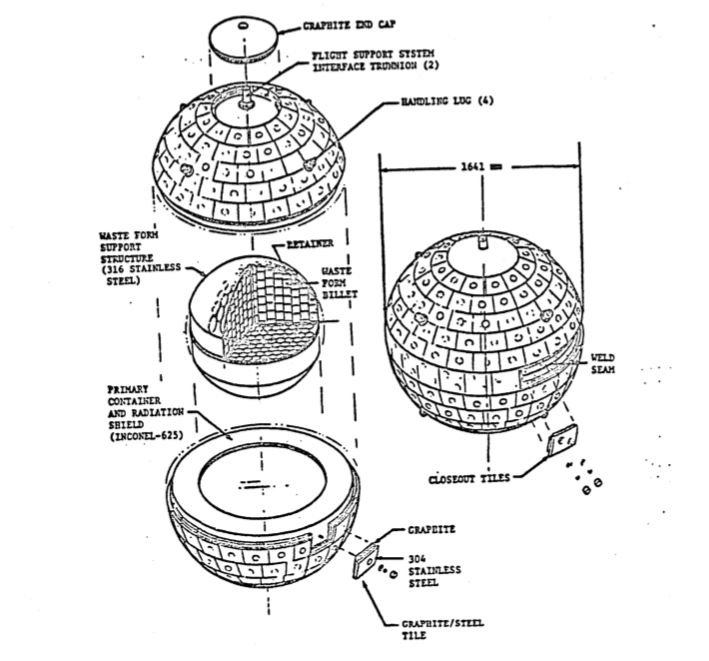
Much like the first proposal, this one also determined that all risks could be mitigated to dispose of spent nuclear fuel into space safely. However, the new proposal called for different criteria to qualify eligible nuclear waste for spaceflight. First, the spent fuel had to be “aged’ at least fifty years from the time it was removed from the reactor. Then it would be processed to remove 95% of the cesium and strontium; those elements would remain on Earth to be stored underground. The extracted plutonium and uranium would be mixed into a compound called cermet. Cermet was a ceramic metallic material that the plutonium and uranium would combine with to form solid bars. Those bars would be inserted into a stainless-steel sphere, transported in a Nuclear Regulatory Commission approved casket to the launch pad to be loaded into the shuttle’s cargo bay.
It’s getting more complicated
So now you might be wondering what next? As you know, this plan never took off, and with good reason. For all the studies that concluded that the idea of shooting nuclear waste into space was a safe disposal method, the most recent study saw one flaw in the plan. That flaw had to do with the primary vehicle carrying that dangerous cargo, the Space Shuttle.
Five years before the Challenger accident, and with one year of experience operating the Space Shuttle, the study concluded that the shuttle would need to be redesigned to increase the safety margin. This early thinking on making the shuttle safer and better would be echoed after the shuttle program ended. A look back at the Space Shuttle successes and failures had many thinking that the shuttle should have always been treated as an experimental spacecraft. Tested, refined, and newer designs built to leverage the learnings. It would never come to pass. Vehicles built with a maximum ten-year lifespan flew for thirty years with modifications to fix inherent flaws, but not to fundamentally redesign the spacecraft.
The requirements outlined to improve the shuttle to make it safe to carry nuclear waste focused on the solid rocket boosters (SRBs). In a foretelling of tragedy to come, the study recommended that the shuttle’s SRBs be replaced with liquid-fueled boosters. It would be a failure in the left solid rocket that would cause the loss of Space Shuttle Challenger and its seven crew members. The risk of potential failure in solid rockets was well known. NASA had initially estimated that the odds were 10,000 to 1 that a solid rocket would fail. However, the Air Force experience was quite different. Nearly one out of every seventy-one launched had a solid rocket motor explode. With the Space Shuttle carrying two, NASA would revise the odds that one in thirty-five launches might expect a failure. As Challenger proved, the odds were not that good.
Did we say more complicated?
Redesigning the shuttle stack would be a costly endeavor. Too expensive for the cash strapped NASA to handle. In addition to refitting the shuttle with liquid-fueled boosters, the shuttles themselves would require modifications. Those modifications were frightening as they alluded to the possibility of an abort or accident with the waste payload onboard. The modifications included:
- The ability to jettison the shuttle’s payload doors, or cycle them open and closed quickly.
- The installation of a blast wall to protect the shuttle crew compartment in the event of an emergency jettison of the payload with the ability for the shuttle to maintain structural integrity following such an event.
- Ability for the crew to escape in the event of an emergency.
- A destruct system in the orbiter to prevent a high-speed impact.
- Strengthening of the orbiter and its landing gear to survive an emergency payload ejection and an abort return with the heavy payload still onboard.
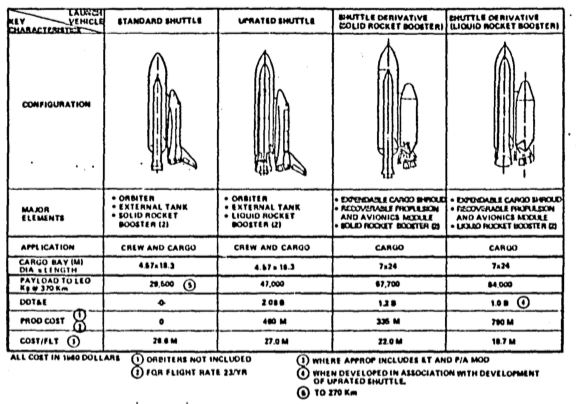
However, it wasn’t the only shuttle recommendation as part of this new plan. The study also proposed introducing the Shuttle Derived Cargo Vehicle. This uncrewed “flying cargo bay” would leverage the shuttle’s external tank and booster configuration. It would be utilized in a mission profile where two shuttle launches were required. The new liquid booster equipped shuttle would carry the nuclear waste payload while the cargo only shuttle would carry an insertion module. The two would rendezvous in space where the shuttle would deploy the payload, back off from the radioactive waste module where it would be remotely mated to the Solar Orbital Insertion Stage (SOIS) using an orbital transfer vehicle carried in the shuttle. The combined vehicle would now launch the payload into a heliocentric orbit between Earth and Venus. The orbital transfer vehicle would be recovered back in the shuttle cargo bay for reuse. The Solar Orbital Insertion Stage would also return to low Earth orbit approximately 165 days after the payload’s initial deployment. It would also be captured by the Space Shuttle on a future mission and returned for reuse. Of course, nothing could be expected to work 100% of the time. So added into the program would be a “rescue capability” should a payload fail to be inserted into a safe orbit.
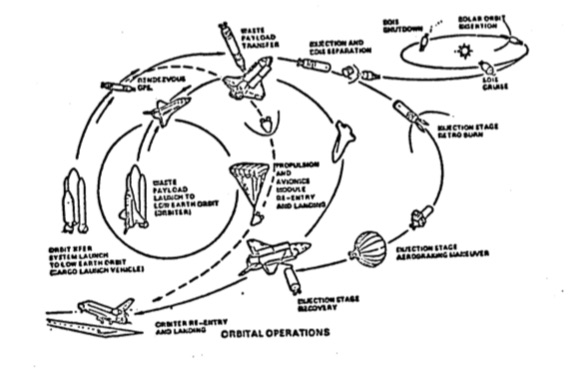
The mission profile would be the same regardless of whether the shuttle was used in a single launch scenario or a dual-launch. The dual launch provided the capability for heavier payloads to be carried aloft. Multiple options were considered for the Solar Orbit Insertion Stage from solar-powered, single and multi-stage boosters, both expendable and reusable. In the end, costs drove the decision, and recovering the stage and returning it to Earth was the most economical.
After all the studies that concurred that the dangerous byproducts of nuclear power could be launched into space on a largely unproven crewed space vehicle was safe, it would be costs that would keep the idea grounded. NASA and the government were in no position to make changes to the costly and fledgling shuttle program. The shuttle was already struggling to keep its existing launch commitments. A quick turnaround between flights and a sustained and easily repeatable flight schedule never materialized. The shuttle was always hampered with delays. In its first three years of operation, the shuttle managed just nine flights. The Challenger accident would forever bust the myth of ensuring that all risks of launching nuclear waste into space could be mitigated. Following Challenger, the shuttle would launch no more than eight times in any single year. Nowhere near the forecasted hundred flights a year that the original study expected would be needed. While Challenger was the only accident to occur after liftoff and before orbit, there were only close calls that would have doomed this idea had they become more publicly known at the time.
After Challenger, a shuttle would carry a nuclear payload into space. The robotic probe Galileo was launched on October 18, 1989, aboard the Space Shuttle Atlantis. While Galileo’s mission was to explore Jupiter and its moons, the shuttle was front and center as the main point of concern for another accident and the potential impact on the environment and the population. Protests threatened to stop the launch. A planned Galileo launch before Challenger had been delayed several times. Those delays put the mission on the schedule just two months after Challenger. The accident would cause a further delay and add to the concern about launching the nuclear payload on a shuttle.
Galileo would be delayed again due to the shuttle grounding after the accident, but that wasn’t the only concern. The probe was scheduled to use a controversial Centaur stage to boost it directly from Earth orbit to Jupiter. At the same time, the Centaur booster was also deemed safe for spaceflight on a crewed vehicle. It, too, had raised concerns that it was an accident waiting to happen. By the time Galileo would fly on the shuttle, the Centaur had been replaced with a less powerful, but safer solid rocket motor. This change would negate the direct Earth to Jupiter path and require multiple gravity assists, including two close passes by Earth before heading to Jupiter. Ironically, causing the same alarm that was first raised that nuclear material could intercept the Earth and rain a fallout over a populated area. NASA had spent a decade and invested fifty million dollars ensuring that the payload was secure. The agency put the odds that a shuttle accident would cause a fallout of Galileo’s fifty pounds of plutonium at 1 in 2500 while anti-nuclear groups placed it at 1 in 430.
Is the idea finally dead and buried?
In hindsight, the initial idea’s complexity and the understated risk seemed so implausible that it was surprising that it got any real consideration. That a study was worth undertaking with an untested and design compromised space vehicle speaks more about the aura of invincibility that shrouded the space program after winning the space race. That aura was expected to carry over into the shuttle program, which allowed the idea of using it to truck nuclear waste into space akin to curbside garbage disposal. In fact, after two accidents, NASA revised its estimate that those first nine shuttle flights in years one through three of the program had a one in nine chance of catastrophic failure. It was an idea that was doomed from the start. If the previous pattern of complex space objectives held true, the costs would run well beyond the study’s most optimistic projections.
While we might think the idea is relegated to collecting dust in the NASA archives, it might still have its day. The problem still exists. Recently, the U.S. Government settled a lawsuit brought by the State of South Carolina regarding nuclear waste in the form of plutonium stored at the Savannah River Nuclear Site. The federal government was required to pay 600 million dollars and agree to remove the 9.5 metric tons of plutonium by 2037. The starting cost of a SpaceX Falcon Heavy launch starts at $90 million and can cost up to $150 million. It can lift eight metric tons to a Geosynchronous Transfer Orbit, about five metric tons to Mars. With the Falcon’s safety record and its use of liquid-fueled boosters, it is not out the question that somewhere someone isn’t thinking about giving it another shot.
— The End —
Copyright 2020 Michael Patrick O’Hara, All Right Reserved
Resources used:
Boeing (1981), “Analysis of Space Systems Study For the Disposal of Nuclear Waste – Executive Summary Volume 1”, D180-26426-1, Boeing Aerospace Company
Broad, William E. (October 10, 1989), “Groups Protest Use of Plutonium on Galileo”, New York Times, https://www.nytimes.com/1989/10/10/science/groups-protest-use-of-plutonium-on-galileo.html
Coates, James, O’Shea, James (February 12, 1986), “NASA IGNORED ODDS: REPORT”, Chicago Tribune, https://www.chicagotribune.com/news/ct-xpm-1986-02-12-8601110466-story.html#:~:text=The%20odds%20of%20a%20solid,NASA%20revised%20its%20failure%20estimates.
Rice, Eric E., Denning, Richard S., Friedlander, Alan L. (February 28, 1972), “Preliminary Risk Assessment For Nuclear Waste Disposal In Space”, Battelle, Columbus Laboratories
Rom, Frank E. (April 11, 1973), “Summary Of The Study Of Disposal Of Nuclear Waste Into Space“, NASA TM X- 68235, NASA Lewis Research Center